The flexible research AFM
Overview
FlexAFM — The flexible research AFM
The FlexAFM has been the instrument of choice for hundreds of researchers in need of a reliable and versatile atomic force microscope system. Built with ease of use and configurability in mind, the capabilities of the FlexAFM cover most research needs: Configure your FlexAFM for 2D materials research in a glovebox, or integrate it with your inverted optical microscope for biological applications - no matter what your research goals are, the Nanosurf FlexAFM provides you with an affordable mid-range instrument that you can expand and upgrade as your requirements evolve.
Reliable and robust
Flexure-based scanner provides flat and linear scanning
Versatile
Flexible sample size and weight thanks to tip-scanning technology
Configurable to your needs
From basic research to advanced experiments: Electrical modes; spectroscopy; motorization and automation; inverted optical microscope integration for bio-research and FluidFM; highly suitable for glovebox use
Optional upgrade to CX Controller:
Additional user input/output channels
More flexibility
Performance boost
Future-proof
Compatibility with DriveAFM accessories: Easy upgrade to high-end
User-friendly handling, light and robust design
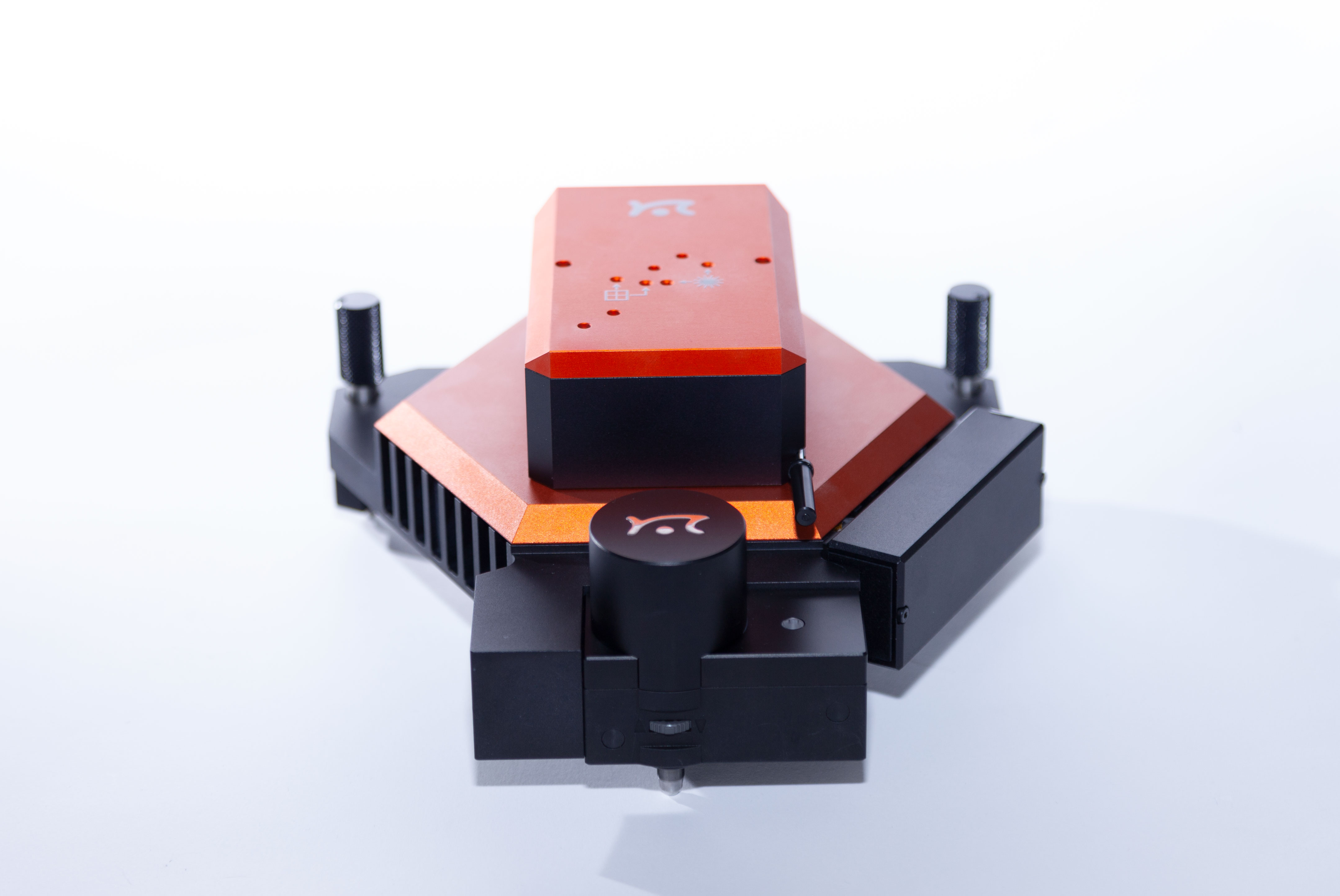
Download the FlexAFM Brochure
Get a printable PDF of the FlexAFM brochure.

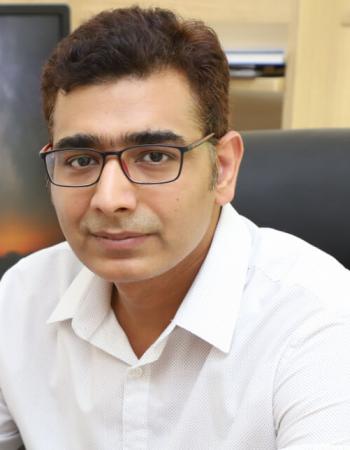
We have been using the FlexAFM system for the last couple of years. The ease of use and its robustness helped us publish a number of articles in high impact journals. I'm very pleased with the performance of your AFM system and would highly recommend your systems for other customers in India and abroad. The technical support from Switzerland as well as local support in India is found to be very satisfactory.
Department of Materials Science & Engineering
INDIAN INSTITUTE OF TECHNOLOGY DELHI
Practical details that really matter in daily use
Cantilever holders with alignment structures are available for use with cantilevers containing alignment grooves. This provides micrometer repositioning accuracy, circumventing laser alignment and allowing you to find the same sample features again and again. Cantilevers enter the image from top to bottom, so sample orientation is always the same, no matter whether you look by eye, CCD camera, or AFM (when scanned at the default scan angle).
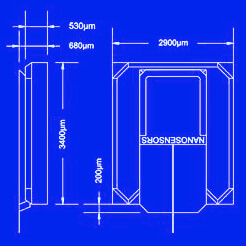

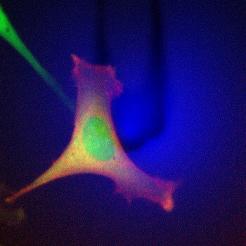
FlexAFM imaging modes
This overview shows which modes the instrument is capable of. Some modes may require additional components or software options. For details, please view the brochure or contact us.
Standard imaging modes
Static Force Mode
Lateral Force Mode
Dynamic Force Mode (Tapping Mode)
Phase Imaging Mode
Thermal imaging modes
Scanning Thermal Microscopy (SThM)
Magnetic properties
Magnetic Force Microscopy
Electrical properties
Conductive AFM (C-AFM)
Piezoelectric Force Microscopy (PFM)
Electrostatic Force Microscopy (EFM)
Kelvin Probe Force Microscopy (KPFM)
Scanning Spreading Resistance Microscopy (SSRM)
Scanning Microwave Microscopy (SMM)
Mechanical properties
Force Spectroscopy
Force Modulation
Stiffness and Modulus
Adhesion
Unfolding and Stretching
Force Mapping
Other measurement modes
Lithography and Nanomanipulation
Electrochemical AFM (EC-AFM)
Scan head dimensions*
The FlexAFM scan head has the same footprint as the DriveAFM: it is compatible with all DriveAFM stages.

*since 2023
Life science
Versatility and performance for biology and life science
For success in life science research, scientists depend on professional tools that can readily provide the information needed, regardless of the tasks at hand. By combining key technologies and components, Nanosurf has made the FlexAFM system one of the most versatile and flexible AFM systems ever, allowing a large variety of biological and life science applications to be handled with ease. With the FlexAFM, you can combine the liquid AFM imaging, spectroscopy and nanomanipulation capacity of this system with the high-end optical techniques available for inverted microscopes.
Flexible system design for life science research
FlexAFM comes with manual and motorized stages for seamless integration on Zeiss, Olympus, Nikon and Leica inverted microscopes or with standalone stages. On the inverted microscope, optical and AFM data can be correlated, as shown here for internal limiting membrane (ILM) of the human retina.
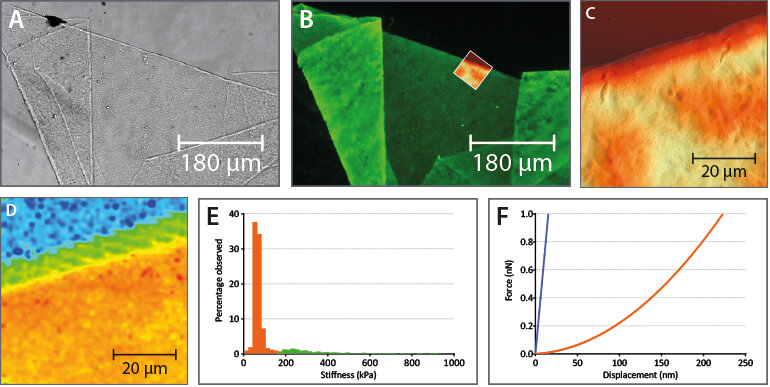
The modular stage, cantilever holder, and software concept allows an easy upgrade of the system to access many new possibilities in life science and materials research. Flex-FPM for cell and nano-manipulation, for example, and Flex-ANA for automatic nanomechanical analysis. In addition, advanced modes like MFM and KPFM that were originally developed for the Flex-Axiom system, are also available for FlexAFM. For measurements that don’t need optical access from below, e.g. for the imaging and spectroscopy of samples like bacteriorhodopsin, a standalone stage makes the FlexAFM compatible with the Nanosurf Isostage and Acoustic Enclosure 300, and generally makes the system much more compact.
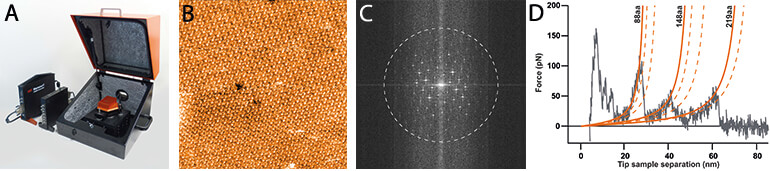
FlexAFM lifescience application examples
Imaging of type I collagen fibrils
Collagen is the most abundant protein in mammals and contributes to more than 25% of the whole-body protein content. It is the main structural protein of the extracellular matrix of connective tissues and provides e.g. tendons and bone with their tensile strength. Most of the collagen found in mammals is fibrillar type I collagen. Type I collagen fibrils show a typical periodic morphology, the so-called D-banding. D-bands result from staggered self-assembly of individual collagen molecules into larger fibrils with a periodicity of about 67 nm.
Images of collagen fibrils from rat tendon were recorded in Prof. Snedeker's research group at the ETH Zürich. One of the reasearch areas of Prof. Snedeker is tendon mechanics and biology.
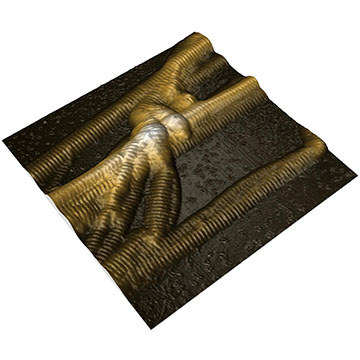
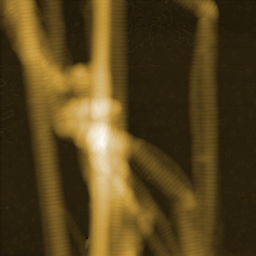

The 3D representation of the AFM topography image nicely shows the typical periodic D-banding of type I collagen on all fibrils. The colloagen topography was recorded in static mode using a Nanosensors PPP-XYCONTR cantilever. AFM images were processed using Nanosurf Report Software. Preparation and imaging of collagen fibrils was performed by Massimo Bagnani, Prof. Snedeker research group, Uniklinik Balgrist, Institute for Biomechanics, ETH Zürich, Switzerland.
Measurements on living cultured cells
Mechanobiology is an emerging research area that deals with the effect of changing physical forces or changes in the mechanical properties of cells and tissues. Several diseases, such as fibrosis and atherosclerosis are associated with changes in tissue stiffness. Moreover, in cancer, the metastatic potential of cancer cells depends on their elastic modulus.
Here, the elastic modulus of living cells from a human breast basal epithelial cell line was measured using a Nanosurf FlexAFM system with the Flex-ANA software.
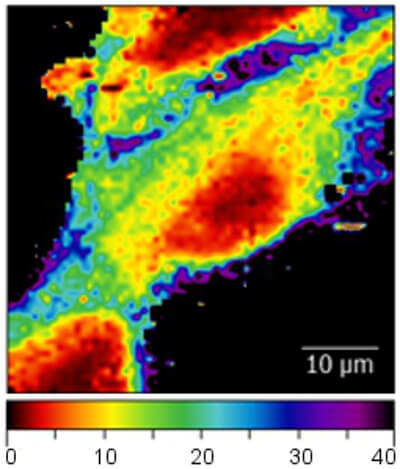

The first image shows the elastic modulus (in kPa) recorded on living breast epithelial cells immersed in cell culture medium. Differences in the elastic modulus within the cell can be clearly observed. The dark area surrounding the cells originate from the much stiffer cell culture dish substrate.
The second image shows the unperturbed cell topography extracted from the force mapping data. The topography is determined from the contact point of each force curve and thus shows the cell topography at zero applied force.

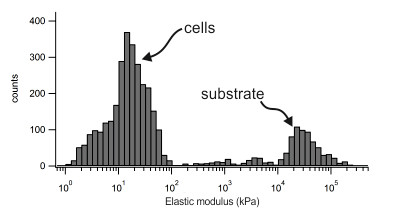
Mapping the elastic modulus data to the 3D topography allows relating the information of both channels. The 3D image was generated using Gwyddion software.
The last image shows the distribution of the elastic moduli extracted from nanomechanical force mapping experiments. The peak at lower moduli corresponds to the stiffness of the cells. The peak at the right originates from the cell culture substrate and shows much higher elastic moduli.
AFM data courtesy of Philipp Oertle, Biozentrum, University Basel.
Single molecule force spectroscopy of bacteriorhodopsin
The force-distance curve below reports the controlled C-terminal unfolding of a single bacteriorhodopsin (BR) membrane protein from its native environment, the purple membrane from Halobacterium salinarium.
Solid and dashed orange lines represent the WLC curves corresponding to the major and minor unfolding peaks observed upon unfolding BR, respectively. The contour length of the stretched polypeptides of the major unfolding peaks is given in amino acids (aa).

This data was recorded using a (10-µm; version 3) in combination with the C3000 controller and a cantilever with a nominal spring constant of 0.1 N/m (Uniqprobe, qp-CONT, Nanosensors).
FlexAFM image gallery
Materials science
The most flexible atomic force microscope for materials research
For success in materials research studies, scientists depend on professional tools that can readily provide the information needed, regardless of the tasks at hand. By advancing key technologies and designs, Nanosurf has made the FlexAFM one of the most versatile and flexible AFMs ever, allowing a large variety of materials research applications to be handled with ease. In combination with the powerful C3000i controller, complex material characterizations are possible.
"The FlexAFM has given us a streamlined and consistent method to cut graphene and graphite gates with ultra-fine precision, allowing for entirely new types of heterostructure devices. What's most remarkable is that this functionality is built in, and is much more simple to setup and use compared to similar techniques in other AFM systems with a much steeper price tag."
J. Ehrets, Kim Lab, Harvard University
The precision and performance you need for your research
The FlexAFM uses an extremely linear electromagnetic scanner for XY movement. This scanner delivers an average linearity deviation of less than 0.1% over the full scan range, top-ranking on the AFM market. The Z-axis is piezo-driven, with a position sensor that enables closed-loop operation. A sensitive cantilever detection system can measure well into the MHz frequency range. The scan head is connected to the full-featured, 24-bit C3000i controller with digital feedback and 2 dual-channel lock-in amplifiers.
Topography of SrTiO3 in dynamic mode
Strontium titanate (SrTiO3, STO) is an oxide of titanium and strontium exhibiting a perovskite structure. It has interesting and partly unique material properties. It is used as substrate for growth of oxide-based thin films and high-temperature superconductors.
STO forms surfaces that show a layered structure. The thickness of individual layers is in the range of a few Angstrom. Atomic force microscopy is an ideal tool to image and measure these structures.


The sample clearly shows the typical layer structure STO. Here, the layers are not perfectly smooth, but exhibit residual roughness of approx. 125 pm (RMS). This is caused by a non-ideal termination process during the preparation of this STO sample.
The graph shows the profile of the image shown above along a line extending from the top left to the bottom right corner of the imaged area. The profile also clearly shows the layered structure of the sample and reveals step heights of approx. 4 Å. Similarly in the right panel, the height distribution histogram of the image above clearly shows approx. 4 Å-spaced peaks for the different layers of the sample.
The FlexAFM has given us a streamlined and consistent method to cut graphene and graphite gates with ultra-fine precision, allowing for entirely new types of heterostructure devices. What's most remarkable is that this functionality is built in, and is much more simple to setup and use compared to similar techniques in other AFM systems with a much steeper price tag.
Kim Lab
Harvard University, MA, USA
Topography and KPFM of CVD grown molybdenum disulfide monolayers
In this application note, monolayer MoS2 grown by chemical vapor deposition (CVD) was imaged with Kelvin probe force microscopy (KPFM) using a FlexAFM to study the contact potential difference variation on a single crystal.
Monolayers of MoS2 were grown on a silicon substrate by chemical vapor deposition (Sample courtesy: University of Illinois – Urbana-Champlain).
Non-uniformity of the contact potential signal across the monolayer can inform about doping profiles and other surface defects.
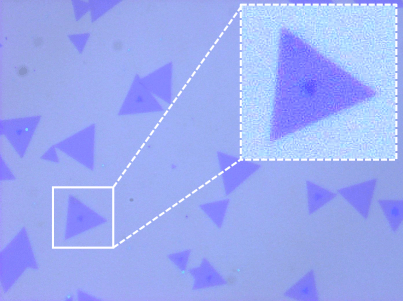
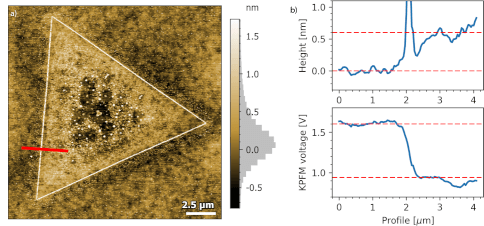
b) Height (top) and KPFM voltage (bottom) profile across monolayer
Measurements using the FlexAFM show a step height of 0.6 nm for the MoS2 monolayer. Concurrent KPFM measurements show a 650 mV contact potential difference between the monolayer and the SiO2 substrate.

All measurements were performed using a FlexAFM system equipped with a ANSCM-PA cantilever from AppNano. Images were processed using MountainsMap SPM.
For more information contact our application scientists
KPFM and MFM on stainless steel
In the experiment shown below, KPFM and topography data were recorded in a single run using a FlexAFM system. Also see the related .
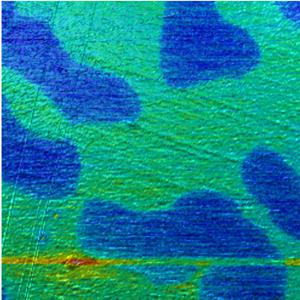
Scan size: 80 µm x 80 µm
Potential range: 200 mV
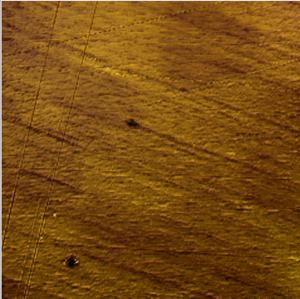
Scan size: 80 µm x 80 µm
Height range: 50 nm
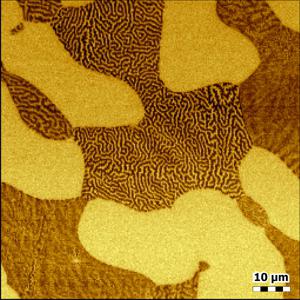
Scan size: 80 µm x 80 µm
Phase range: 10°
FlexAFM image gallery
FluidFM®
FluidFM®: The established microfluidic tool for single-cell biology and nanomanipulation
FluidFM literally brings microfluidics to the tip of an AFM cantilever. This way, it combines the microfluidics with the force sensitivity and positional accuracy of an AFM allowing a range of exciting applications in single-cell biology and nanoscience.
Nanosurf has a long experience providing AFM systems with FluidFM having launched the FlexAFM with FluidFM in 2011 together with Cytosurge. This integration of FluidFM on Nanosurf platforms has grown over time and now the FluidFM technology is available for Nanosurf's FlexAFM, CoreAFM and DriveAFM platforms.

A tool for research pioneers
- A tool to conduct original research at the frontiers of science from bacterial adhesion to cell-cell interaction and from spotting to injection and extraction.
- A solution with optical, force, and fluidic control.
- FluidFM can also be combined with PicoBalance to measure the mass of cells and microparticles picked up by the FluidFM probe.
FluidFM application examples with FlexAFM
Cell-cell adhesion forces
FluidFM cell adhesion measurements were extended to study cell-cell interaction. This can be the force between a cell (on the cantilever) and a cell below on a substrate (fig. 1 A), but also between a cell and its surrounding cells in a confluent layer (fig. 1 B).
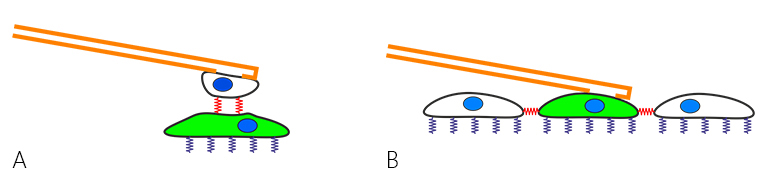
Dr. Noa Cohen of Prof. Tanya Konry's group at Northeastern university in Boston studied cell-cell adhesion forces with a Flex-FPM system to gain more insight into tumor progression and metastasis [Cohen et al. (2017)]. Figure 2 shows an optical image of the method depicted in fig. 2 A that was used by Cohen for this study.

Data courtesy of Tanya Konry group, Northeastern University, Boston, USA.
Single MCF7 breast cancer cell were immobilized on the cantilever. The cell was then pushed on different cell types that were immobilized on a substrate. The measured cell adhesion between MCF7 cancer cells to different cell types were found to develop differently with incubation time. In these experiments, the reversible binding of cells allowed the different cell pairs to be studied with the same probe (fig. 3), allowîng better comparison of measurements.
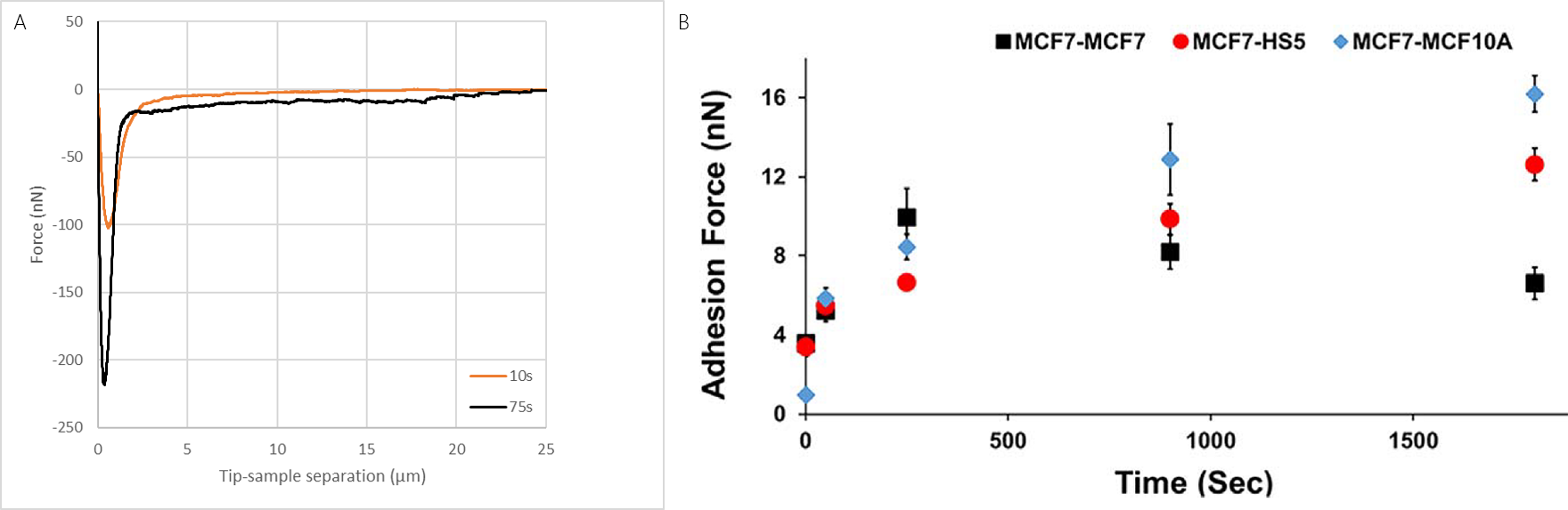
Dr. Ana Sancho from the group Prof. Jürgen Groll's group at the University of Würzburg extensively studied the interaction between a cell and its neighbors in a confluent layer of epithelial cells (fig. 2 B) [Sancho et al. (2017)]. Fig. 4 shows the cantilever picking up a cell from a confluent layer (A). After removal the empty space from where the cell was picked up is visible (B). Again, the epthelial cells of interest could be selected based on cell size and cell shape using the inverted microscope.
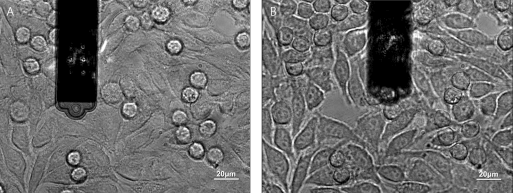
Human endothelial cells from the umbilical artery were found to exert strong intercellular forces (figures 6 A & B). The forces could be decreased significantly by overexpression of the so-called Muscle Segment Homeobox 1 (MSX1). The MSX1 induces a endothelial-to-mesenchymal transition. This transition is a process involved in cardiovascular development and disease. Complementary to these adhesion experiments, the Flex-FPM system was also used to perform nano-indentation measurements. To this end colloidal beads aspired to the cantilever and force curves were recorded on the cells.

Both examples strongly benefitted from FluidFM™ technology provided by the Flex-FPM solution. In case of the confluent layer the large forces of up to over 1.5µN eliminate chemical binding to study cell-cell adhesion forces. In both cases the reversible binding provided the experiments with the necessary speed-up to obtain sufficient statistics.
Spotting and lithography with FluidFM
In cell biology, micropatterning is becoming a widely accepted technique to address cellular behavior at the single-cell level. The ability to guide cell growth by local suppression or stimulation, for example, is beneficial for studying cell growth, for developing cell-based sensors, and for tissue engineering applications. The production of microfabricated biosensors is another application that requires precise placement of biomaterials on a substrate.
FluidFM® technology by Cytosurge enables deposition of (bio)molecules and particles at defined locations with micrometer accuracy and with femtoliter volumes. The closed channel is capable of depositing molecules from a liquid in both air and liquid environment. This enables numerous applications in biomedicine, cell- and microbiology, as well as non-biological nanolithography.
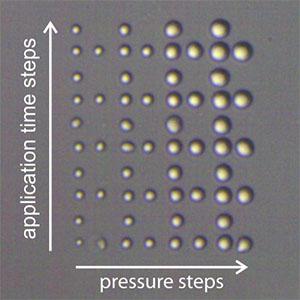
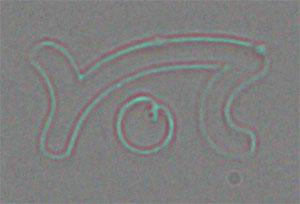

Download the FluidFM brochure for more information
ANA
ANA: The perfect tool for fully automated nanomechanical data acquisition and analysis
The ANA system is an automated solution for AFM-based nanomechanical analysis. It is designed to investigate the nanomechanical properties of materials such as cells, tissues, scaffolds, hydrogels, and polymers on multiple or large samples via force spectroscopy and force mapping in an intuitive and automated fashion.
ANA is compatible with Nanosurf's FlexAFM scan heads: for materials science, as well as for life science applications on a setup on an inverted microscope.
I really enjoy using the Flex-ANA for its excellent force mapping capabilities on polymer materials. The scientists at Nanosurf are highly knowledgeable and responsive, and have developed an easy-to-use system with many features (both in hardware and software) purposefully designed for force curve measurements.
From automated calibrations and programmable stages to streamlined integration of multiple data sets, the Flex-ANA offers important benefits for any research involving force curve measurements.
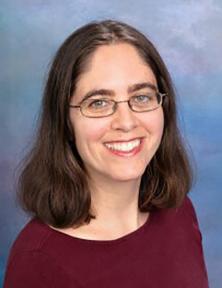
SurfaceChar
Nanomechanical analysis of large or multiple samples
The combination of a straight-forward workflow-based experimental procedure in the ANA software, carefully selected hardware elements and motorization, and well-designed and thought-through control algorithms are hallmarks of the ANA setup on FlexAFM. Measurement locations on large or multiple samples are defined by simply clicking on an overview image with a large field-of-view that covers the entire accessible 32 mm × 32 mm sample area. Height differences of up to 5 mm can be easily overcome by the system, and many types of nanomechanical measurements, analyses and models can be performed and used!
Handle demanding samples with ease
During an experiment, the ANA system coordinates the movement of the scan head and the motorized XYZ translation stage (range: 32 mm × 32 mm × 5 mm) to cope with large sample height variations. The system can optionally be equipped with an additional 100-µm Z piezo for an extended force spectroscopy range. Thus, samples with macroscopic and microscopic roughness in the range of several millimeters and micrometers, respectively, as well as soft and sticky samples, can be easily addressed.
Learn about details in our webinar by Dr. Edward Nelson
Learn about the automated nanomechancial analysis module and its application in analyzing blended polymers. See the ease of use in data collection and analysis, and how automated collection of data from multiple samples has the potential to save time and make your research more efficient.
Operation by experts as well as novice users
The ANA control software provides a Basic and an Expert user mode that are designed for different degrees of user experience and training:
In Basic mode, less experienced users are guided through the setup and calibration of the ANA system step-by-step. Optimized workflows guarantee that everything is done in the correct sequence, that nothing is forgotten, and that all is set properly.
In Expert mode, experienced users have more freedom in operating and setting up the system to suit their individual needs. Measurement parameters can also be prepared and stored by an expert, and then subsequently used by less experienced users in Basic mode.
The ANA software was partly developed within the framework of the OYSTER project, which has received funding from the European Union’s Horizon 2020 research and innovation program under grant agreement No 760827.

To learn more about ANA, please visit the ANA product page.
Specifications
FlexAFM specifications and dimensions
System specifications
FlexAFM 5 scan head specifications with C3000i controller | 100-µm scan head | 10-µm scan head |
---|---|---|
Sample size | Unlimited without sample stage 100 mm on sample stage | |
Maximum Petri dish height (fluid level) | 9 mm (6 mm) | |
Manual height adjustment range | 6 mm | |
Motorized approach range (at tip position) | 2 mm | |
Max. scan range (XY) | 100 µm1 | 10 µm1 |
Max. height range (Z) | 10 µm2 | 3 µm1 |
XY-linearity mean error | < 0.1% | |
XY-flatness at maximum scan range | typ. 5 nm | typ. 1 nm |
Detector bandwidth | DC - 4 MHz | |
Detector noise level (RMS) | typ. 60 pm / max. 100 pm (3, 4) | |
Z-sensor noise level (RMS) | typ. 180 pm / max. 200 pm (3) | |
Z-measurement noise level (RMS, static mode in air) | typ. 100 pm / max. 200 pm | |
Z-measurement noise level (RMS, dynamic mode in air) | typ. 35 pm / max. 50 pm | |
Scan head dimensions | 413 x 158 x 53 mm | |
Scan head weight | 1.25 kg | |
(1) Manufacturing tolerances ± 5% (2) Manufacturing tolerances ± 10% (3) Measured at 2 kHz (4) Measured with XYContr cantilever |
C3000i controller — Core hardware specifications | |
---|---|
X/Y/Z-axis scan and position controller | 3× 24-bit DAC (200 kHz) |
X/Y/Z-axis position measurement | 1× 24-bit ADC (200 kHz) |
Excitation & modulation outputs | 2× 16-bit DAC (20 MHz) |
Analog signal input bandwidth | 0–5 MHz |
Main input signal capturing | 2× 16-bit ADC (20 MHz) 2× 24-bit ADC (200 kHz) |
Additional user signal outputs | 1× 24-bit ADC (200 kHz) |
Digital synchronization | Sync Out 1/2: digital outputs, signal range 0/5V TTL pulses |
FPGA module and embedded processor | ALTERA FPGA, 32-bit NIOS-CPU, 80 MHz, 256 MB RAM, multitasking OS |
Communication | USB 2.0 Hi-Speed to PC and scan head interface |
System clock | Internal quarts (10 MHz) or external clock |
Power | 90–240 V AC, 70 W, 50/60Hz |
Cantilever | |
---|---|
Width | min. 20 μm |
Length | min. 40 μm |
Reflective coating | Reflective coating recommended |
Liquid measurements | Yes, with gold coating |
Alignment grooves | Required by default Special cantilever holders without alignment grooves are available |
Resonance frequency dynamic mode Easyscan 2 Controller | 15 kHz to 350 kHz |
Resonance frequency dynamic mode C3000 Controller | < 4 MHz |
Cantilever shape | Single rectangular cantilevers and multilever cantilevers (depending on scan head version and cantilever holder) |
Chip thickness | 300 μm, 500 μm or 600 μm depending on cantilever holder |
Scan head dimensions
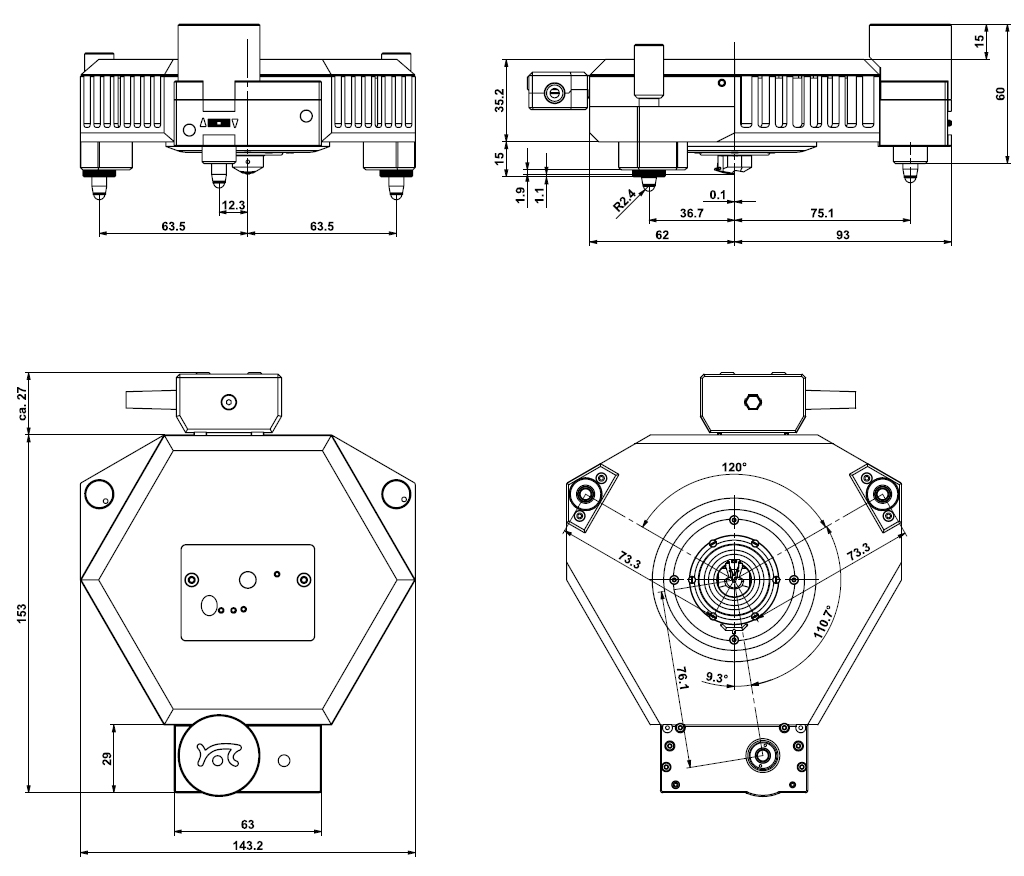